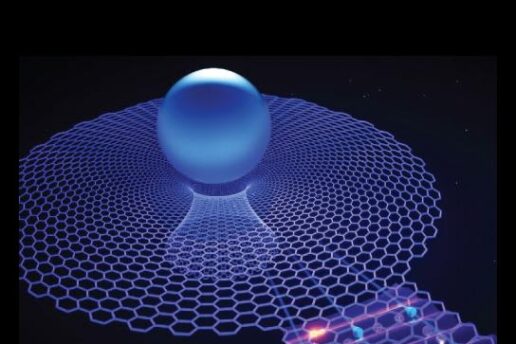
Physicists Show That a “Quasiparticle” Made of Light and Matter Can Be Dragged by a Current of Electrons
Work could impact optical computing, nanolasers, more
Light was thought to move at a fixed rate until 1851, when a French physicist—the first to accurately clock the speed of light—showed it could also be slowed or accelerated simply by shining a light beam with or against the flow of moving water. Decades later, Einstein seized on Hippolyte Fizeau’s landmark water-pipe experiments in developing his theory of relativity.
Now, new research in Nature led by physicists at MIT and Columbia University shows that a quasiparticle made of waves of photons and electrons—a plasmon polariton—has a similar ability to change speeds when immersed in an electrical current flowing through a sheet of graphene. But there’s a hitch: the polaritons appear to more easily shift gears in one direction—to a slightly slower speed—when traveling against the flow of electrons.
The finding is a big deal for plasmonics, a field with a rock-star name dedicated to finding new and efficient ways of controlling light down at the nearly invisible scale of individual atoms. Polaritons have two perks. Their relatively slow speed compared to photons makes them a good proxy for manipulating light. Polariton waves are also minuscule; dozens can squeeze into the wavelength of one photon.
“Our work enables one to carry out relativistic experiments in a simple tabletop setting and opens the door to studies of nonequilibrium light-matter interaction at the nanoscale,” says Denis Bandurin, an MIT Pappalardo Fellow in Physics and a corresponding author of the Nature paper. “Our results may also find some applications for nanophotonics thanks to a property known as non-reciprocity. This property is usually hard to achieve in optical experiments, but it is induced in graphene due to the discovered plasmonic Fizeau drag.”
The leader of the project (and the other corresponding author of the paper), is Dmitri Basov. Basov, the Higgins Professor of Physics at Columbia, has devoted most of his lab to studying the behavior of polaritons. “They possess the best virtues of electrons and photons,” he says. “They’re compact but still quantum, which means they can be manipulated on ultra-fast time scales.”
Two MIT faculty are also authors of the paper.
“This is an interesting case study of motional coupling between two very different collective flows, the collective plasma oscillations and the DC current. Remarkably, plasma oscillations are found to acquire a nonreciprocal (one-way) character in the presence of the flow, preserving a high degree of optical coherence. As such, plasmonic Fizeau effect is of interest for the quest for new effects due to the electron-electron interactions, and new transport phenomena due to such effects, says Professor of Physics Leonid Levitov.
Comments Pablo Jarillo-Herrero, the Cecil and Ida Green Professor of Physics, “This is one of those rare occasions where we were able to realize a beautiful seminal classical experiment but in a much more quantum mechanical way, by using a combination of photons and electrons–and using an electrical current in our favorite 2D material–graphene! And of course, the results came with a nice surprise, a diode-like effect!”
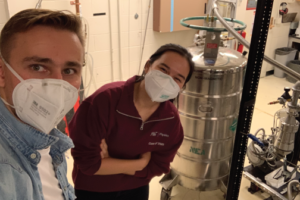
In addition to Bandurin, Levitov, and Jarillo-Herrero, other authors from MIT are Undergraduates Isabelle Phinney and Haoyang Gao (Phinney has since graduated); and Graduate Student Zhiyu Dong. (Jarillo-Herrero and Levitov are also affiliated with MIT’s Materials Research Laboratory.)
In addition to Basov, other authors from Columbia are Yinan Dong, Lin Xiong, Zhiyuan Sun, R. Jing, Alex .S. McLeod, Shuai Zhang, Frank Ruta, R. Pan, and Andrew J. Millis.
Other authors are Song Liu and James Edgar of Kansas State University and Michael M. Fogler of the University of California, San Diego.
An Unexpected Result
In the Nature study, Bandurin, Basov and colleagues recreated Fizeau’s experiments on a speck of graphene made up of a single layer of carbon atoms. Hooking up the graphene to a battery, they created an electrical current reminiscent of Fizeau’s water streaming through a pipe. But instead of shining light on the moving water and measuring its speed in both directions, as Fizeau did, they generated an electromagnetic wave with a compressed wavelength—a polariton—by focusing infrared light on a gold nub in the graphene. The activated stream of polaritons looks like light but polaritons are physically more compact due to their short wavelengths.
The researchers clocked the polaritons’ speed in both directions. When they traveled with the flow of the electrical current, they maintained their original speed. But when launched against the current, they slowed by a few percentage points.
“We were surprised when we saw it,” says Bandurin. “First, the device was still alive, despite the heavy current we passed through it—it hadn’t blown up. Then we noticed the one-way effect, which was different from Fizeau’s original experiments.”
The researchers repeated the experiments over and over, led by the study’s first author, Yinan Dong. Finally, it dawned on them. “Graphene is a material that turns electrons into relativistic particles,” Dong says. “We needed to account for their spectrum.”
‘The Enablers of Nano-optics’
A group at Berkeley Lab found a similar result, published in the same issue of Nature. Beyond reproducing the Fizeau effect in graphene, both studies have practical applications. Most natural systems are symmetric, but here, researchers found an intriguing exception. Basov said he hopes to slow down, and ultimately, cut off the flow of polaritons in one direction. It’s not an easy task, but it could hold big rewards.
“Engineering a system with a one-way flow of light is very difficult to achieve,” said Milan Delor, a physical chemist working on light-matter interactions at Columbia who was not involved in the research. “As soon as you can control the speed and direction of polaritons, you can transmit information in nanoscale circuits on ultrafast timescales. It’s one of the ingredients currently missing in photon-based circuits.”
Plasmonics researchers are also excited about the detailed images to come out of the experiments. They show that polaritons can serve as nanoscale probes, they say, triggering and recording electron-electron interactions in a system. This information provides clues about how graphene and other quantum materials will behave in the real world.
“The images are effectively a read-out of the material properties of graphene,” Delor says.
“I like to call polaritons the enablers of nano-optics,” says James Schuck, a mechanical engineer and plasmonics researcher at Columbia who was not involved in the work. “They’re useful for probing all sorts of materials at the nanoscale.”
This work was funded by the Department of Energy, an MIT Pappalardo Fellowship, the Office of Naval Research, the Air Force Office of Scientific Research, the Gordon and Betty Moore Foundation, and the National Science Foundation.