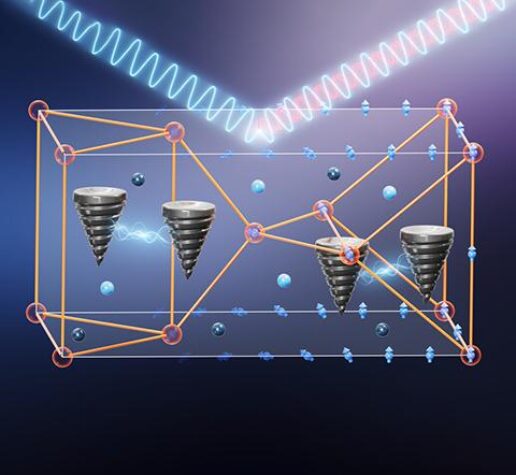
Making more magnetism possible with topology
MIT researchers, including senior Linh Nguyen, graduate student Phum Siriviboon, and alumni Quynh T. Nguyen ’22 and Tongtong Liu PhD ’23, show how topology can help create magnetism at higher temperatures.
Researchers who have been working for years to understand electron arrangement and magnetism in certain semimetals have been frustrated by the fact that the materials only display magnetic properties if they are cooled to just a few degrees above absolute zero.
A new MIT study led by Mingda Li, associate professor of nuclear science and engineering, and co-authored by Nathan Drucker, a graduate research assistant in MIT’s Quantum Measurement Group and PhD student in applied physics at Harvard University, along with Thanh Nguyen and Phum Siriviboon, MIT graduate students working in the Quantum Measurement Group, is challenging that conventional wisdom.
The open-access research, published in Nature Communications, for the first time shows evidence that topology can stabilize magnetic ordering, even well above the magnetic transition temperature — the point at which magnetism normally breaks down.
“The analogy I like to use to describe why this works is to imagine a river filled with logs, which represent the magnetic moments in the material,” says Drucker, who served as the first author of the paper. “For magnetism to work, you need all those logs pointing in the same direction, or to have a certain pattern to them. But at high temperatures, the magnetic moments are all oriented in different directions, like the logs would be in a river, and magnetism breaks down.
“But what’s important in this study is that it’s actually the water that’s changing,” he continues. “What we showed is that, if you change the properties of the water itself, rather than the logs, you can change how the logs interact with each other, which results in magnetism.”
A surprising connection between topology and magnetism
In essence, Li says, the paper reveals how topological structures known as Weyl nodes found in CeAlGe — an exotic semi-metal composed of cerium, aluminum and germanium — can significantly increase the working temperature for magnetic devices, opening the door to a wide range of applications.
While they are already being used to build sensors, gyroscopes, and more, topological materials have been eyed for a wide range of additional applications, from microelectronics to thermoelectric and catalytic devices. By demonstrating a method for maintaining magnetism at significantly higher temperatures, the study opens the door to even more possibilities, Nguyen says.
“There are so many opportunities people have demonstrated — in this material and other topological materials,” he says. “What this shows is a general way that can significantly improve the working temperature for these materials,” adds Siriviboon.
That “quite surprising and counterintuitive” result will have substantial impact on future work on topological materials, adds Linda Ye, assistant professor of physics in Caltech’s Division of Physics, Mathematics and Astronomy.
“The discovery by Drucker and collaborators is intriguing and important,” says Ye, who was not involved in the research. “Their work suggests that electronic topological nodes not only play a role in stabilizing static magnetic orders, but more broadly they can be at play in the generation of magnetic fluctuations. A natural implication from this is that influences from topological Weyl states on materials can extend far beyond what was previously believed.”
Princeton University professor of physics Andrei Bernevig agrees, called the findings “puzzling and remarkable.”
“Weyls nodes are known to be topologically protected, but the influence of this protection on the thermodynamic properties of a phase is not well understood,” says Andrei Bernevig, who was not involved in the work. “The paper by the MIT group shows that short-range order, above the ordering temperature, is governed by a nesting wave vector between the Weyl fermions that appear in this system … possibly suggesting that the protection of the Weyl nodes somehow influences magnetic fluctuations!”
While the surprising results challenge the long-held understanding of magnetism and topology, they are the result, Li says, of careful experimentation and the team’s willingness to explore areas which otherwise might go overlooked.
“The assumption had been that there was nothing new to find above the magnetic transition temperature,” Li explains. “We used five different experimental approaches and were able to create this comprehensive story in a consistent way and put this puzzle together.”
Putting together the clues
To demonstrate the presence of magnetism at higher temperature, the team began by combining cerium, aluminum, and germanium in a furnace to form millimeter-sized crystals of the material.
Those samples were then subjected to a battery of tests, including thermal and electrical conductivity tests, each of which revealed a clue to the material’s unusual magnetic behavior.
“But we also undertook some more exotic methods to test this material,” Drucker says. “We hit the material with a beam of X-rays which was calibrated to the same energy level as the cerium in the material, and then measured how that beam scattered.
“Those tests had to be done in a very large facility, in a Department of Energy national lab,” he continues. “Ultimately, we had to do similar experiments at three different national labs to show that there is this hidden order there, and that’s how we found the strongest evidence.”
Part of the challenge, Nguyen says, is that conducting such experiments on topological materials is typically very difficult to do and usually provides only indirect evidence.
“In this case, what we did was conduct several experiments using different probes, and by putting them all together, that gives us a very comprehensive story,” he says. “In this case it’s five or six different clues, and a big list of instruments and measurements that played into this study.”
Opening the door to future studies
Going forward, Li says, the team plans to explore whether the relationship between topology and magnetism can be demonstrated in other materials.
“We believe this principle is general,” he says. “So we think this may be present in many other materials, which is exciting because it expands our understanding of what topology can do. We know it can play a role in increasing conductivity, and now we’ve shown it can play a role in magnetism as well.”
Additional future work, Li says, will also address possible applications for topological materials, including their use in thermoelectric devices which convert heat into electricity. While such devices have already been used to power small devices, like watches, they are not yet efficient enough to provide power for cellphones or other, larger devices.
“We have studied many good thermoelectric materials, and they are all topological materials,” Li says. “If they can show this performance with magnetism … they will unlock very good thermoelectric properties. For example, this will help them to run at a higher temperature. Right now, many only run at very low temperatures to collect waste heat. A very natural consequence of this would be their ability to work at higher temperatures.”
Building a better understanding of topological materials
Ultimately, Drucker says, the research points to the fact that, while topological semimetals have been studied for a number of years, relatively little is understood about their properties.
“I think our work highlights the fact that, when you look over these different scales and use different experiments to study some of these materials, there are in fact some of these really important thermoelectric and electrical and magnetic properties that start to emerge,” Drucker says. “So, I think it also gives a hint not only towards how we can use these things for different applications, but also towards other fundamental studies to follow up on how we can better understand these effects of thermal fluctuations.”
This work was supported by funding from the U.S. Department of Energy, Office of Science, Basic Energy Sciences; the National Science Foundation (NSF) Designing Materials to Revolutionize and Engineer our Future Program; and an NSF Convergence Accelerator Award.