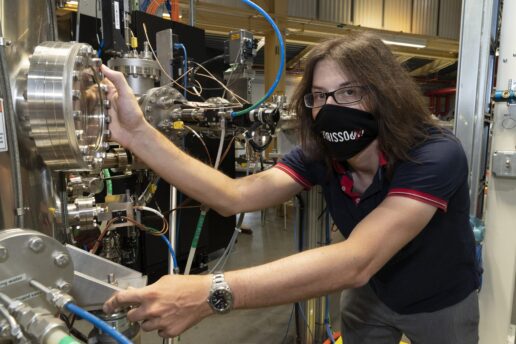
Physicists uncover secrets of world’s thinnest superconductor
First experimental evidence of spin excitations in an atomically thin material helps answer 30-year-old questions, could lead to better medical diagnostics and more.
Physicists from across three continents report the first experimental evidence to explain the unusual electronic behavior behind the world’s thinnest superconductor, a material with myriad applications because it conducts electricity extremely efficiently. In this case, the superconductor is only an atomic layer thick.
The work, led by an MIT professor and a physicist at Brookhaven National Laboratory, was possible thanks to new instrumentation available at only a few facilities in the world. The resulting data could help guide the development of better superconductors. These in turn could transform the fields of medical diagnostics, quantum computing, and energy transport, which all use superconductors.
The subject of the work belongs to an exciting class of superconductors that become superconducting at temperatures an order of magnitude higher than their conventional counterparts, making them easier to use in applications. Conventional superconductors only work at temperatures around 10 kelvins, or -442 degrees Fahrenheit.
These so-called high-temperature superconductors, however, are still not fully understood. “Their microscopic excitations and dynamics are essential to understanding superconductivity, yet after 30 years of research, many questions are still very much open,” says Riccardo Comin, the Class of 1947 Career Development Assistant Professor of Physics at MIT. The new work, which was reported recently in Nature Communications, helps answer those questions.
Comin’s colleagues on the work include Jonathan Pelliciari, a former MIT postdoc who is now an assistant physicist at Brookhaven National Laboratory and lead author of this study. Other authors are Seher Karakuzu and Thomas A. Maier of Oak Ridge National Laboratory; Qi Song, Tianlun Yu, Xiaoyang Chen, Rui Peng, Qisi Wang, Jun Zhao, and Donglai Feng of Fudan University; Riccardo Arpaia, Matteo Rossi, and Giacomo Ghiringhelli of Politecnico di Milano (Arpaia is also affiliated with Chalmers University of Technology); Abhishek Nag, Jiemin Li, Mirian García-Fernández, Andrew C. Walters, and Ke-Jin Zhou of Diamond Light Source in the United Kingdom; and Steven Johnston of the University of Tennessee at Knoxville.
World’s thinnest superconductor
In 2015 scientists discovered a new kind of high-temperature superconductor: a sheet of iron selenide only one atomic layer thick capable of superconducting at 65 K. In contrast, bulk samples of the same material superconduct at a much lower temperature (8 K). The discovery “sparked an investigative flurry to decode the secrets of the world’s thinnest superconductor,” says Comin, who is also affiliated with MIT’s Materials Research Laboratory.
In a regular metal, electrons behave much like individual people dancing in a room. In a superconducting metal, the electrons move in pairs, like couples at a dance. “And all these pairs are moving in unison, as if they were part of a quantum choreography, ultimately leading to a kind of electronic superfluid,” says Comin.
But what is the interaction, or “glue,” that holds these pairs of electrons together? Scientists have known for a long time that in conventional superconductors, that glue is derived from the motion of atoms within a material. “If you look at a solid sitting on a table, it doesn’t appear to be doing anything,” Comin says. However, “a lot is happening at the nanoscale. Inside that material, electrons are flying by in all possible directions and the atoms are rattling; they’re vibrating.” In conventional superconductors, the electrons use the energy stored in that atomic motion to pair up.
The glue behind electrons’ pairing in high-temperature superconductors is different. Scientists have hypothesized that this glue is related to a property of electrons called spin (another, more familiar property of electrons is their charge). The spin can be thought of as an elementary magnet, says Pelliciari. The idea is that in a high-temperature superconductor, electrons can pick up some of the energy from these spins, known as spin excitations. And that energy is the glue they use to pair up.
Until now, most physicists thought that it would be impossible to detect or measure spin excitations in a material only an atomic layer thick. That is the remarkable achievement of the work reported in Nature Communications. Not only did the physicists detect spin excitations, but, among other things, they also showed that the spin dynamics in the ultra-thin sample were dramatically different from those in the bulk sample. Specifically, the energy of the fluctuating spins in the ultra-thin sample was much higher — by a factor of four or five — than the energy of the spins in the bulk sample.
“This is the first experimental evidence of the presence of spin excitations in an atomically thin material,” says Pelliciari.
State-of-the art equipment
Historically, neutron scattering has been used to study magnetism. Since spin is the fundamental property of magnetism, neutron scattering would appear to be a good experimental probe. “The problem is that neutron scattering doesn’t work on a material that is only one atomic layer thick,” says Pelliciari.
Enter resonant inelastic X-ray scattering (RIXS), a new experimental technique that Pelliciari helped pioneer.
He and Comin discussed the potential for using RIXS to study the spin dynamics of the new ultra-thin superconductor, but Comin was initially skeptical. “I thought, ‘Yes, it would be great if we could do this, but experimentally it’s going to be next-to-impossible,’” Comin remembers. “I thought it was a true moonshot.” As a result, “when Johnny collected the very first results, it was mind-blowing for me. I’d kept my expectations low, so when I saw the data, I jumped on my chair.”
Only a few facilities in the world have advanced RIXS instruments. One, located at Diamond Light Source (UK) and led by Zhou, is where the team conducted their experiment. Another one, which was still being built at the time of the experiment, is at Brookhaven National Laboratory. Pelliciari is now part of the team running the RIXS facility, known as the Beamline SIX, at the National Synchrotron Light Source II located at Brookhaven Lab.
“The impact of this work is two-fold,” says Thorsten Schmitt, head of the Spectroscopy of Novel Materials Group at the Paul Scherrer Institut in Switzerland, who was not involved in the work. “On the experimental side, it is an impressive demonstration of the sensitivity of RIXS to the spin excitations in a superconducting material only an atomic layer thick. Furthermore, the [resulting data] are expected to contribute to the understanding of the enhancement of the superconducting transition temperature in such thin superconductors.” In other words, the work could lead to even better superconductors.
Valentina Bisogni, lead scientist for the Beamline SIX who was not involved in this study, says, “the understanding of unconventional superconductivity is one of the main challenges faced by scientists today. The recent discovery of high-temperature superconductivity in a monolayer-thin film of iron selenide renewed the interest into the iron selenide system, as it provides a new route to investigate the mechanisms enabling high-temperature superconductivity.
“In this context, the work of Pelliciari et al. presents an enlightening, comparative study of bulk iron selenide and monolayer-thin iron selenide revealing a dramatic reconfiguration of the spin excitations,” Bisogni says.
This research was supported by the U.S. Air Force Office of Scientific Research, the MIT-POLIMI Program (Progetto Rocca), the Swiss National Science Foundation, the U.S. Department of Energy (DOE), the U.S. Office of Naval Research, the Fondazione CARIPLO and Regione Lombardia, the Swedish Research Council, the Alfred P. Sloan Foundation, and the National Natural Science Foundation of China.
This research used resources of the National Synchrotron Light Source II, a DOE Office of Science user facility located at DOE’s Brookhaven Lab.
Paper: “Evolution of spin excitations from bulk to monolayer FeSe”