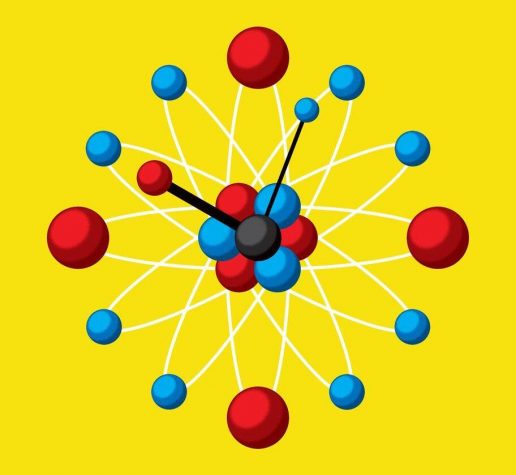
The Clock Paradox of Quantum Physics
How does an unstable nucleus ‘know’ when it’s time to decay? The answer may lie in the fabric of space itself.
In 1935, the physicist Erwin Schrödinger invented a thought-experiment to test whether quantum theory fully describes reality. Known as “Schrödinger’s Cat,” the experiment asks us to imagine a cat in a sealed box, together with a vial containing poisonous gas and a mechanism that can shatter the vial. The mechanism is triggered when an unstable atomic nucleus decays, breaking the glass and killing the cat.
According to quantum theory, however, the nucleus can be in different states, unchanged or decayed, at the same time. Only when it is observed do the possible states “collapse,” making it definite whether the nucleus has decayed or not. Thus the poor cat somehow hovers between life and death, waiting for somebody or something to collapse the nucleus’s state (and thus its own). This weird situation suggested to Schrödinger that quantum theory is missing something. With all respect to him, however, I think that the weird part of the setup isn’t the cat; it’s the behavior of the unstable nucleus.
To see why, consider radioactive dating, a marvelous technique for determining the age of objects. It is used in archaeology, to date human artifacts; in geology, to date stones and strata; and in astrophysics, to date distant stars and gas clouds. The accuracy of radioactive dating is undisputed, yet the principle behind it is deeply strange.
Many objects contain some nuclei that are unstable, meaning that they will eventually decay, changing into a different isotope and emitting energy in the process. In every equal interval of time, a fixed proportion of the unstable nuclei in a sample will decay. The amount of time it takes for half of them to decay is known as the isotope’s half-life.
What makes radioactive nuclei such ideal clocks is that they are reliably unreliable. An isotope’s half-life can be determined accurately by observing lots of decays. For instance, radioactive carbon, which is used to date organic material, has a half-life of about 5,700 years. But it’s impossible to predict when any individual nucleus will decay. In fact, an individual nucleus is a kind of anti-clock: It does not register the passage of time at all. There is no observable difference between old and young nuclei. They remain ideally young, we might say, until they suddenly and explosively die. By monitoring decays within this homogeneous population we measure time statistically, with confidence.
How do such simple things as atomic nuclei, or even more elementary particles like neutrons or muons, “know” their half-life? Where are the springs or pendulums or batteries that keep track of time for them? Strange questions! But the answer supplied by modern physics is stranger. These objects are like hair-trigger bombs in a stormy environment. Space itself, seething with quantum fluctuations, supplies passing gusts, and every so often one is strong enough to trigger an explosion. In this picture, nuclei are basically simple and passive. It is space, saturated with quantum fields, that is complex and active.
Quantum theory does not introduce chance into the description of nature as a theoretical whimsy. Reality, as revealed in the basic facts of radioactivity, simply is weird and chancy, whether we like it or not. By bringing that weirdness within the scope of rational thought, quantum theory can at least domesticate it.
Originally appeared in the December 5, 2020, print edition as ‘The Paradox Of Quantum Clocks.’
Frank Wilczek is the Herman Feshbach Professor of Physics at MIT, winner of the 2004 Nobel Prize in Physics, and author of the books Fundamentals: Ten Keys to Reality (2021), A Beautiful Question: Finding Nature’s Deep Design (2015), and The Lightness of Being: Mass, Ether, and the Unification of Forces (2009).