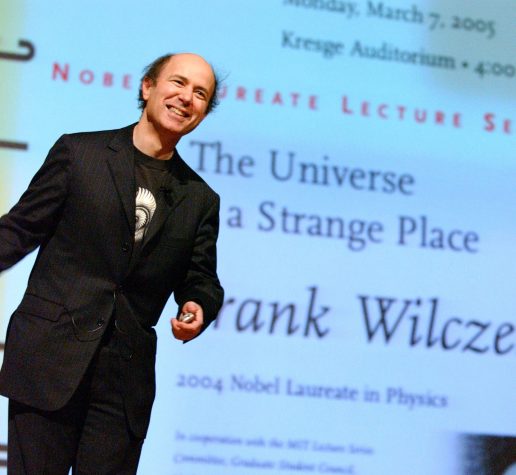
The Quantum Computers in Our Future
New systems using ‘qubits’ instead of classical bits will be vastly more powerful—but they still face important obstacles.
In recent years there has been a lot of progress in the field of quantum computers. IBM, Google and Microsoft are big players, as are several university-based research groups and national laboratories around the world. There is a widespread sense that the technology has great promise, but the exact nature and scope of that promise has been only vaguely defined. Indeed, it’s becoming clear that quantum computers will come in a variety of different sizes and shapes, suited for different purposes.
Ordinary computers store information in the form of bits, which can be in one of two states, 1 or 0. (“Bit” is short for binary digit.) Long sequences of bits can encode complex information of many kinds, from chess positions to protein structures to pictures of cats. Quantum computers, on the other hand, use quantum bits, called qubits. Qubits can be 1, 0 or something in between— so-called superpositions, which are indissoluble mixtures of 1 and 0. In different exploratory technologies, qubits can take the form of individual atoms, electrons, minuscule superconducting loops or more exotic “anyons” (a breed of emergent particle that I studied and named). Qubits, being more intricate than bits, can store information more densely.
Classical computers aren’t well adapted to simulating quantum systems. They simply don’t have enough room to store and manipulate the required information. Quantum computers promise to be much better at quantum mechanics. In principle, that will enable them to calculate the properties of matter, including potential catalysts, drugs, solar cells and batteries, eliminating the need for laborious, hit-or-miss experiments.
Quantum computers are also touted as tools for cracking codes. But those applications are very demanding, requiring precise calculations involving thousands of qubits. That prospect is futuristic. For the foreseeable future, general purpose quantum computers will be restricted to a few tens of qubits, and they will be error-prone.
For while qubits are powerful, they’re also delicate and hard to work with. If qubits are jostled by environmental fields or particles, it can scramble the information they were meant to encode. We’re familiar with the fact that a computer memory can get erased by a large magnetic field, for example, but quantum memory is sensitive to much smaller fields.
Quantum simulators are a less flexible but much easier alternative for studying quantum mechanics. The idea is to mimic the behavior of a particular quantum system that is interesting but difficult to study by using another quantum system that is more user-friendly. Assemblies of ultracold atoms are especially useful quantum simulators, because we can manipulate their interactions by bathing them in adjustable laser light, together with electric and magnetic fields. For example, we can use cold atoms as stand-ins for neutrons, to learn about neutron-star interiors.
Another promising development is classical-quantum hybrids, in which a classical computer can call on a relatively small quantum “coprocessor” to do critical calculations within its own programs. The coprocessor strategy follows the spirit of Graphical Processing Units (GPUs)— superfast chips that do some specialized operations extremely efficiently. GPUs were originally developed for use in computer games, where they make it possible to update fast-paced displays. But ingenious researchers have exploited them in many other ways—notably, to simulate how quarks and gluons build up protons.
The hybrid strategy could start to deliver on the promise of quantum computation for chemistry and materials science long before large, general-purpose quantum computers are available—if they ever are. Bring on the QPUs!
Originally appeared on March 14, 2019 on The Wall Street Journal website as ‘The Quantum Computers in Our Future‘
Frank Wilczek is the Herman Feshbach Professor of Physics at MIT, winner of the 2004 Nobel Prize in Physics, and author of the books Fundamentals: Ten Keys to Reality (2021), A Beautiful Question: Finding Nature’s Deep Design (2015), and The Lightness of Being: Mass, Ether, and the Unification of Forces (2009).