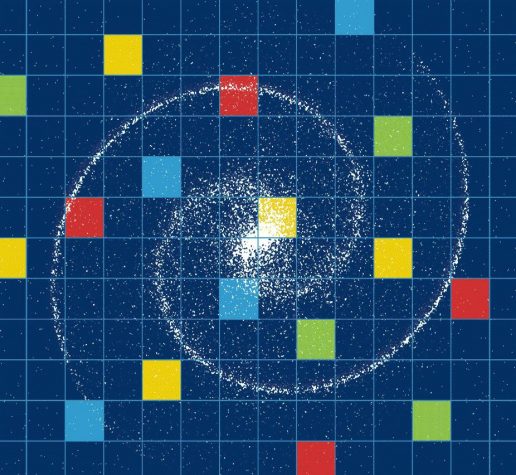
This Yearʼs Nobels Have Cosmic Reach
Prize-winning research shows how the universe evolved from its uniform beginnings to the complexity we observe today.
Last week, the Nobel Prize in Physics went to James Peebles for theoretical contributions to physical cosmology, along with Michel Mayor and Didier Queloz for their work on exoplanets—distant planets that orbit stars other than our sun.
These lines of research both shed light on the universe and our position in it, but they do so from very different perspectives. Modern physical cosmology reveals that the universe started out amazingly simple and homogeneous, while the study of exoplanets reveals that at present it is complex and diverse. That contrast poses a big question: How did the complexity emerge?
Early in the history of the universe, all the matter in it was hot, dense and very nearly uniform. It was also rapidly expanding. Those ideas are the heart of the standard Big Bang model, which lets us account for many observations, including the fact that distant galaxies are moving away from us and the relative abundance of different chemical elements.
Notably, the model predicted the existence of a lingering Big Bang afterglow— the so-called microwave background radiation that fills all space. That afterglow was duly observed, providing a snapshot of the early universe. Mr. Peebles pulled those lines of evidence together into a coherent scenario of the history of the universe and spelled out its consequences for the size, shape and distribution of galaxies.
The early hot gas that filled the universe was completely random in its molecular motions and chemical mixing. It was, to a very good approximation, in the condition physicists call “complete thermal equilibrium.” Ordinarily, once systems reach complete thermal equilibrium they stay there. They remain uniform and featureless; they do not develop structure or “come to life.”
Our universe escaped that dismal fate primarily because gravity, acting over vast reaches of space and time, makes uniformity unstable. Gravity wants things to clump. Thus the material in the universe, at first highly uniform, fragmented under the influence of gravity into vast cloudlike structures.
At first the clouds were tenuous and wispy, but over time, under the continuing influence of gravity, their material condensed further. The matter in the universe gradually evolved into its present configuration: galaxies hosting stars and planets, all separated by yawning regions of nearly empty space.
Planetary matter, cool and dense, then began to host another level of fragmentation and diversification: the emergence of complex chemistry, and even—in at least one case—intelligent life. Because planets are relatively small and emit no light of their own, it is very hard to detect them from far away. Mr. Mayor and Mr. Queloz pioneered the delicate technologies that have quickly taken exoplanetary astronomy from science fiction into a thriving, data-driven enterprise.
This is a very broad-brush account of how the complex universe that we inhabit today could have emerged. Though many crucial details need filling in, the outlines are straightforward and widely accepted: The emergence of abundant complexity from simple beginnings and simple laws takes a long time and requires lots of matter (but maybe nothing else). Thankfully, our universe is blessed with plentiful supplies of both.
Originally appeared on October 17, 2019 on The Wall Street Journal website as ‘This Yearʼs Nobels Have Cosmic Reach‘
Frank Wilczek is the Herman Feshbach Professor of Physics at MIT, winner of the 2004 Nobel Prize in Physics, and author of the books Fundamentals: Ten Keys to Reality (2021), A Beautiful Question: Finding Nature’s Deep Design (2015), and The Lightness of Being: Mass, Ether, and the Unification of Forces (2009).