Cosmology and Astroparticle Physics
The interface between particle theory and early-universe cosmology has been a lively area of research since the 1970’s, when physicists realized that hot big bang cosmology would imply that fundamental properties of our universe — from the abundance of chemical elements to maybe also the density of baryons — were determined by high-energy physics processes in the nascent universe. In the present day, our understanding of the universe seems to require “dark energy” and “dark matter” components, which do not have any simple explanation in the Standard Model of particle physics.
Inflationary cosmology, pioneered by Alan Guth in 1980-81, proposed that early-universe particle physics could be responsible for the production of essentially all the matter in the universe, explaining the uniformity of the universe and predicting its average mass density. It was soon discovered that quantum fluctuations during inflation might be responsible for the ripples in the mass density of the early universe—ripples that formed the seeds for structure formation, and which are now visible in the anisotropies of the cosmic microwave background (CMB). The inflationary prediction of the mass density has now been confirmed to an accuracy of about half a percent, and the patterns of ripples seen in the CMB agree very well with the predictions of simple inflationary models.
At the same time, the CMB and other probes have allowed us to measure the amount of dark matter in the universe to percent-level precision. Physicists have established that dark matter must have mass and exert gravity, but it must also be relatively slow-moving, and its interactions (other than gravity) with known particles must be weak or absent. This leaves open a huge range of possibilities for dark matter, from new particles tens of orders of magnitude lighter than even neutrinos, through to primordial black holes formed in the first instants of the universe’s existence.
Mikhail Ivanov uses cosmological large-scale structure to understand dark matter, dark energy, and inflation. The new generation of galaxy surveys will allow for precision tests of these sectors through their imprints on the observed matter distribution. An accurate theoretical understanding of these imprints will be key to harvesting new cosmological information from large-scale structure in this novel regime of high precision.
The cosmology and particle astrophysics program in the CTP focuses on implications for fundamental physics and applications of field-theoretic techniques, complementing the work of our colleagues in the Laboratory for Nuclear Science and the MIT Kavli Institute for Astrophysics and Space Research.
Research on inflation
David Kaiser and Alan Guth are continuing to pursue the connection between particle theory and early-universe cosmology. Much of the work of Kaiser and his group has centered around understanding the dynamics and the predictions of inflationary models that include realistic features from high-energy physics: multiple interacting fields, each with nonminimal gravitational couplings. His group has also developed novel techniques to study the dynamics of inflationary models before and after inflation, including conditions under which the universe may enter an inflationary phase even amid significant inhomogeneities, and the mechanisms by which inflation ends during the “reheating” epoch, when the universe becomes filled with ordinary matter in thermal equilibrium at a high temperature. Alan Guth and collaborators have recently worked on models of inflation at a very low energy scale, and on the cosmology of axions. Guth’s recent research has also included the study of eternal inflation, with the issues it raises concerning the definition of probabilities. Guth and Kaiser are both working with a group of postdocs, graduate students, and undergraduates to study the possibility of the production of primordial black holes in the context of a particular type of inflation, called hybrid inflation.
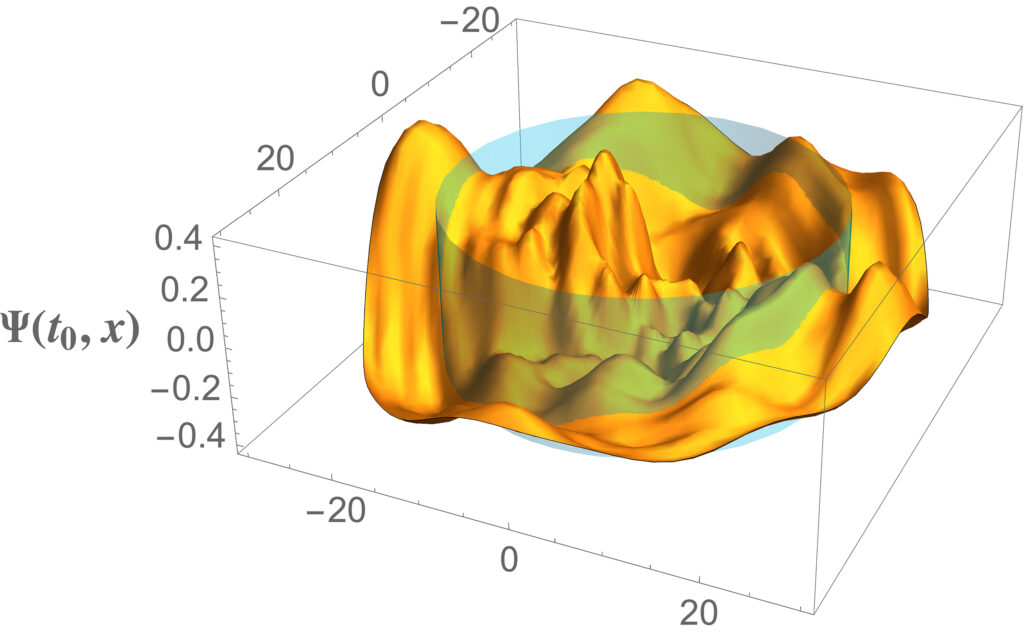
Testing the foundations of quantum mechanics
In a separate line of investigation, David Kaiser and Alan Guth have both been part of the international “Cosmic Bell” collaboration, which has tested the foundations of quantum mechanics by conducting experimental tests of Bell’s inequality using real-time astronomical observations of high-redshift quasars to determine which measurements to perform on entangled particles. Kaiser has also worked with Joseph Formaggio in the Laboratory for Nuclear Science to use data on neutrino oscillations to test the Leggett-Garg inequality across unprecedented length-scales.
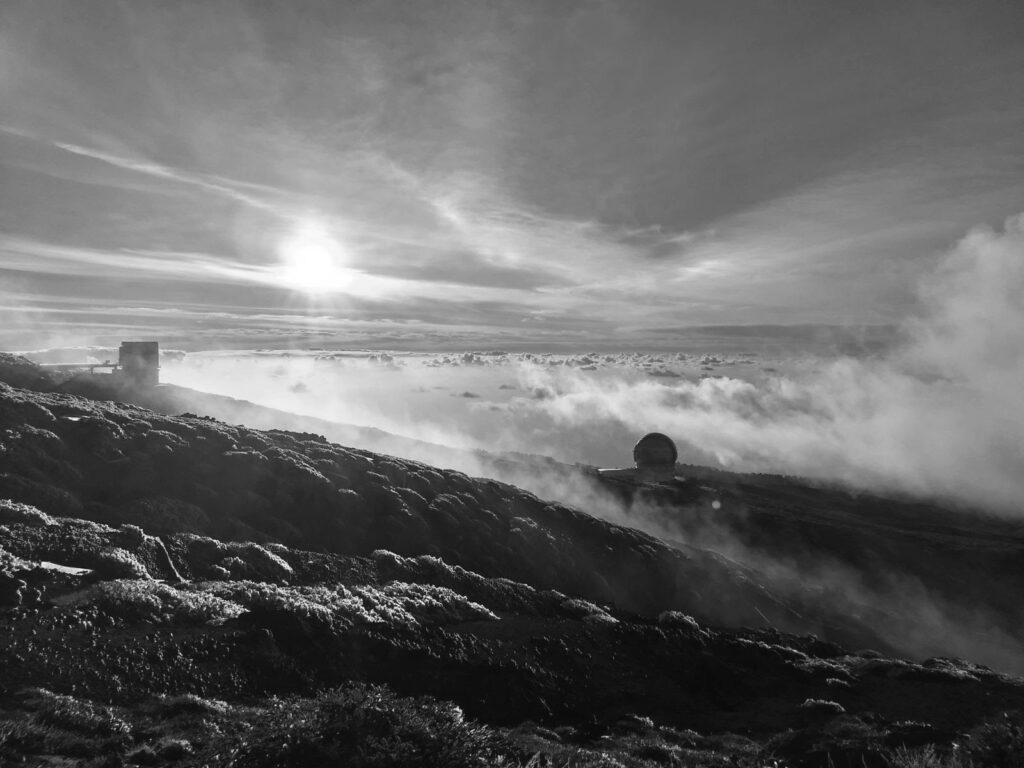
Astrophysical and cosmological dark matter signals
Video by Bill Lattanzi | MIT Center for Theoretical Physics
Annihilations or decays of dark matter could modify the thermal and ionization history of the universe, with possible observational consequences for nucleosynthesis, the cosmic microwave background and the redshifted 21cm line. In the present era, the same phenomena could provide striking signals from regions of high dark matter density. Tracy Slatyer’s group works extensively on the interpretation of such signals, developing new constraints and identifying possible signatures of dark matter physics, from radio to gamma-ray wavelengths, with a particular focus on data from the Fermi Gamma-Ray Space Telescope and signals from the early universe.
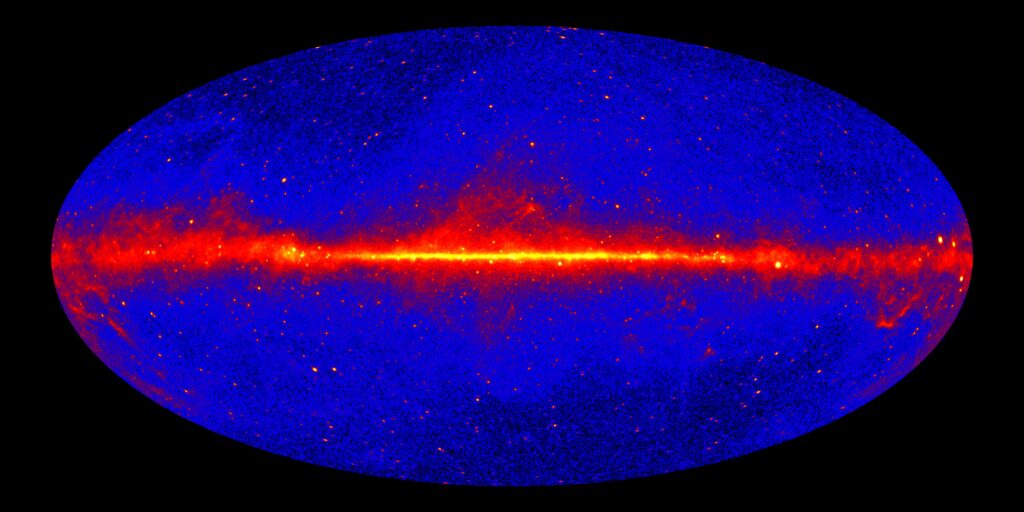